Motivation
Mechanical ventilation is a life-sustaining intervention for patients receiving respiratory support. Modern ICU ventilators offer an array of modalities with widely ranging levels of support, control, and responsiveness.
Our lab seeks to advance the standard of care for mechanical ventilation by designing and evaluating novel strategies for delivering safe and effective ventilation while minimizing risks associated with ventilator-induced lung injury.
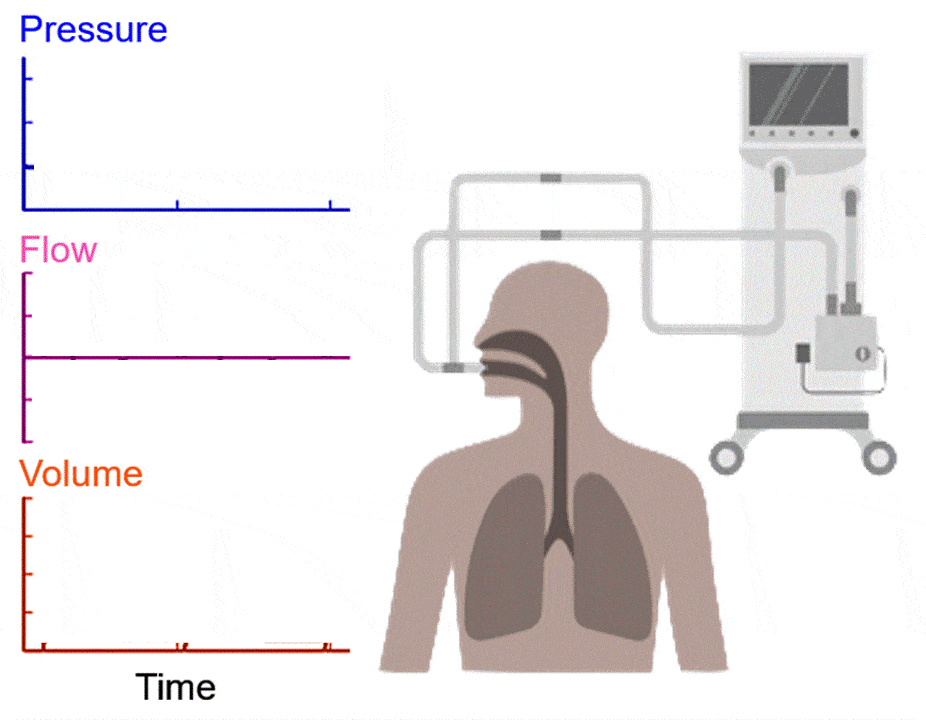
We develop and test novel modalities for respiratory monitoring and control of mechanical ventilation.
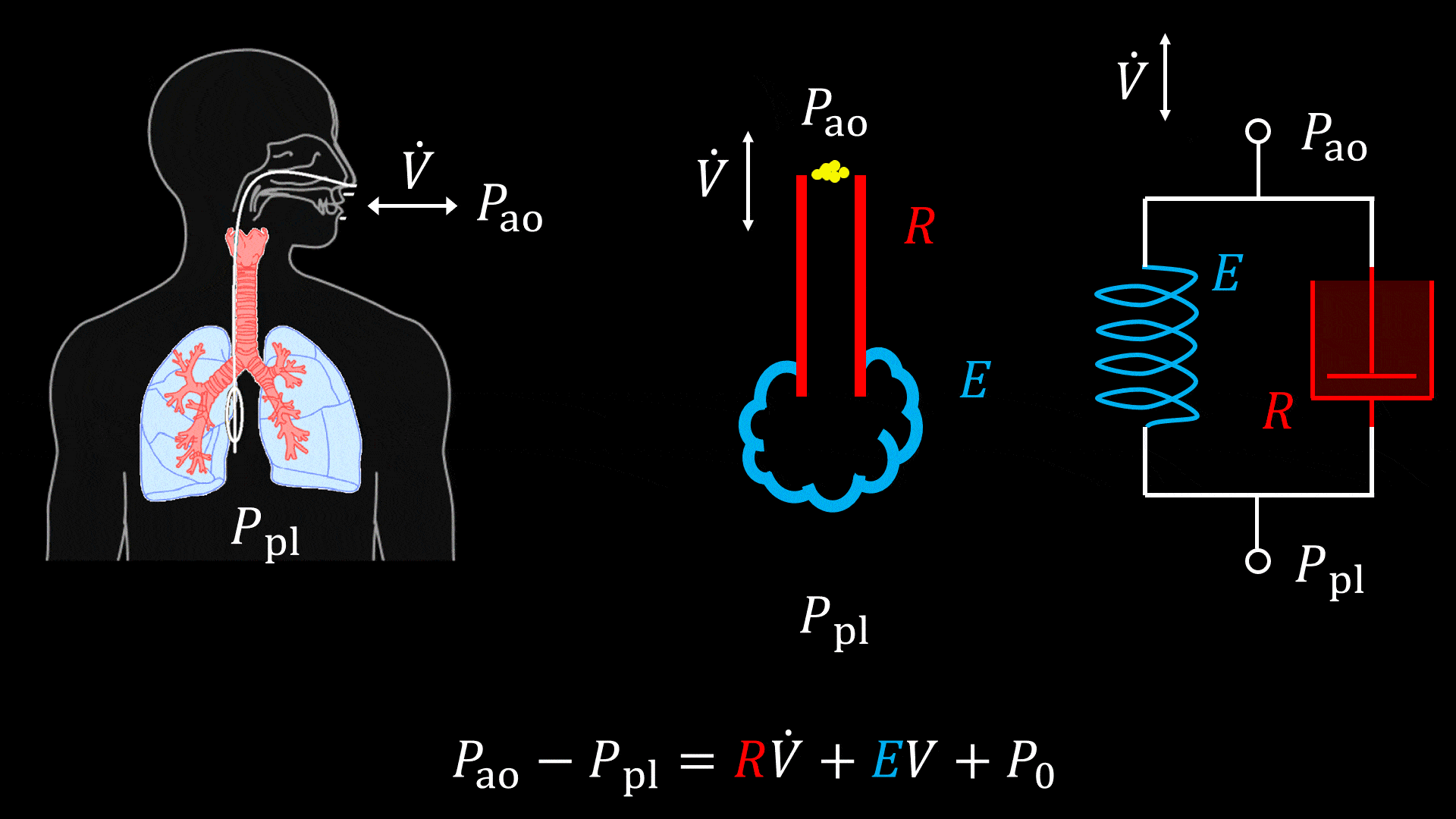
Respiratory Mechanics
The mechanical properties of the lungs determine their response to controlled pressure (Pao), flow (V̇), or volume (V) waveforms at the airway opening, relative to pleural pressure (Ppl).
The simplest lumped-element mechanical model of the respiratory system considers only a single compartment, with a dashpot resistance (R) and a spring elastance (E).
Resistance and elastance parameters can be extracted from pressure, flow, and volume waveforms measured by the mechanical ventilator, using inverse modeling approaches for parameter estimation.
Learn more: Go to journal site
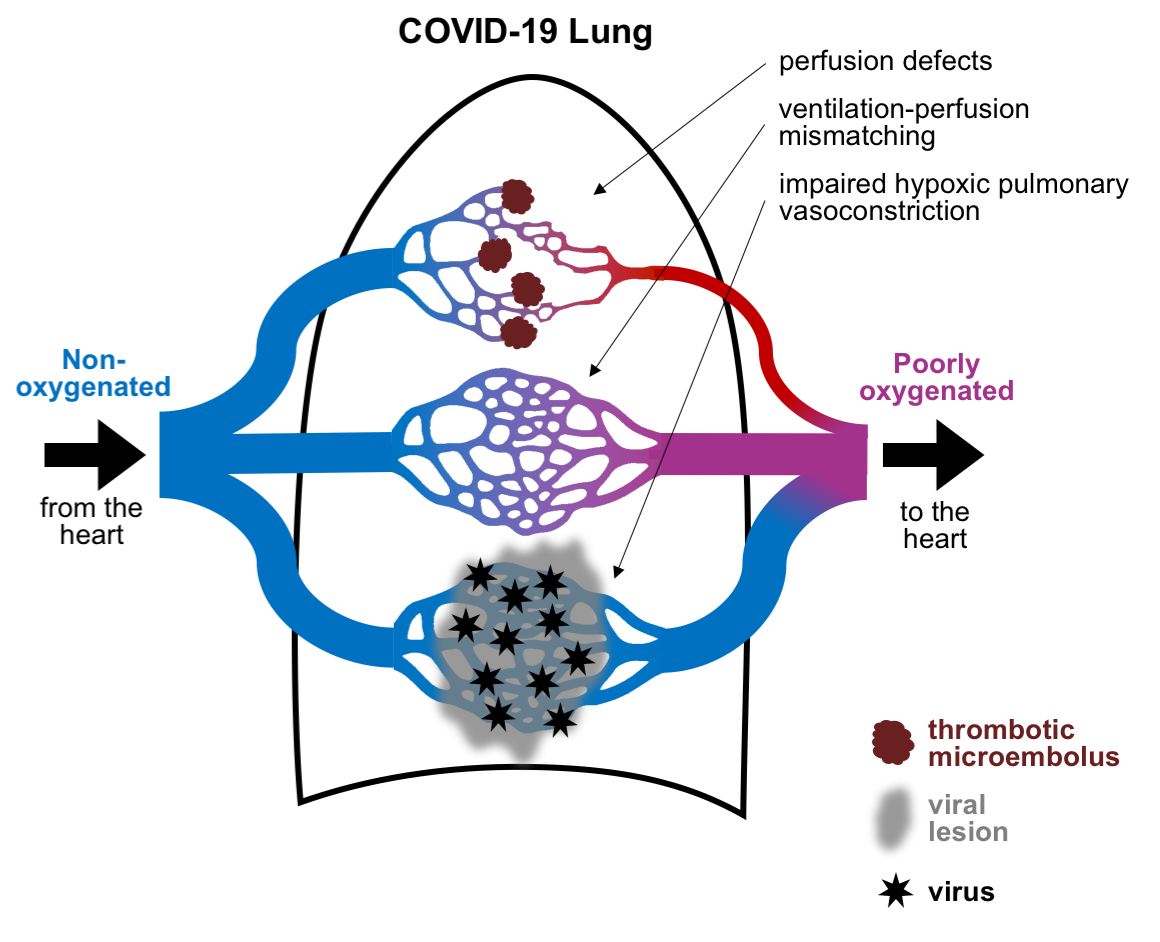
Compartmentalized Gas Exchange
Mathematical models can provide useful physiologic and pathophysiologic insights into disease mechanisms and manifestations. We design simulations of respiratory gas exchange and disease-related impairments (e.g., alterations in blood flow, oxygen diffusion, and ventilation-perfusion matching). These simulations allow us to predict a range of possible patient scenarios, and assess the sensitivity of patient status to variations in model parameters.
Learn more: Go to journal site
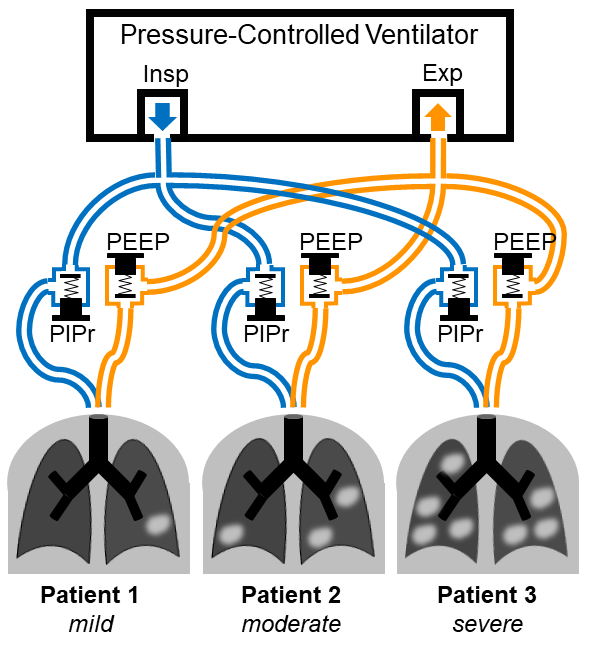
Ventilator-Sharing Risks
Mathematical models can also inform predictions and guidance around atypical or high-risk scenarios of patient care. For example, a surge in demand for mechanical ventilators may elicit attempts at ventilator-sharing across multiple patients simultaneously.
We simulated different approaches to ventilator-sharing via modified breathing circuits, each attempting to individualize ventilation for all co-ventilated patients. This study revealed the potential pitfalls associated with mechanical interdependence between co-ventilated patients, and furthermore highlighted the least dangerous approach which maximized independent control.
Learn more: Go to journal site
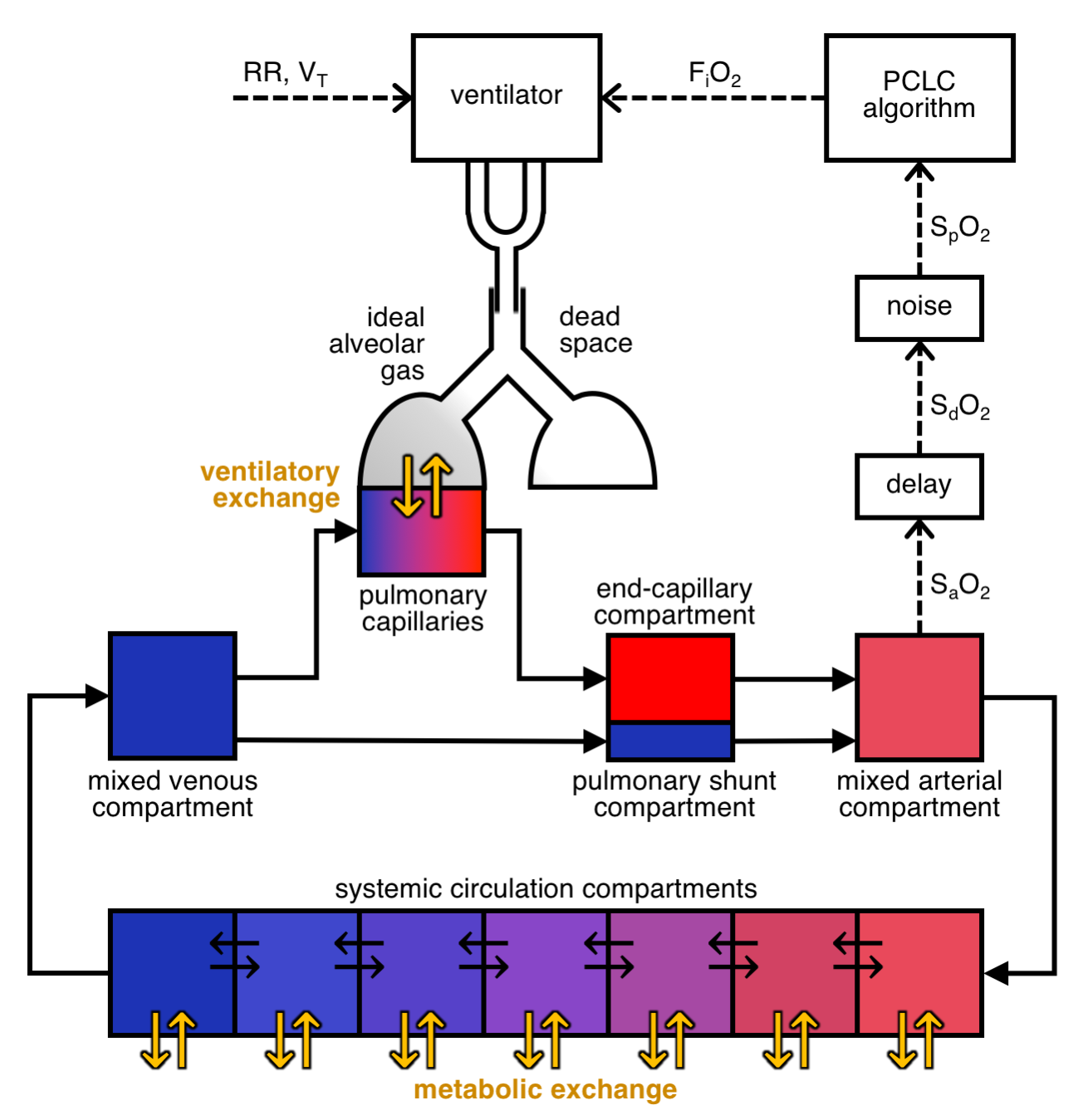
Physiologic Closed-Loop Control
Automating control of the inspired oxygen fraction (FiO2) based on measured peripheral oxyhemoglobin saturation (SpO2) enables the ventilator to respond to changes in patient condition with appropriate ventilation settings, while conserving oxygen resources.
We design computational models to simulate a vast array of patient parameters that might influence the controller's performance. These simulations are used to quantitatively evaluate the safety, efficacy, and stability of the automated algorithms, and iteratively improve upon algorithm design.
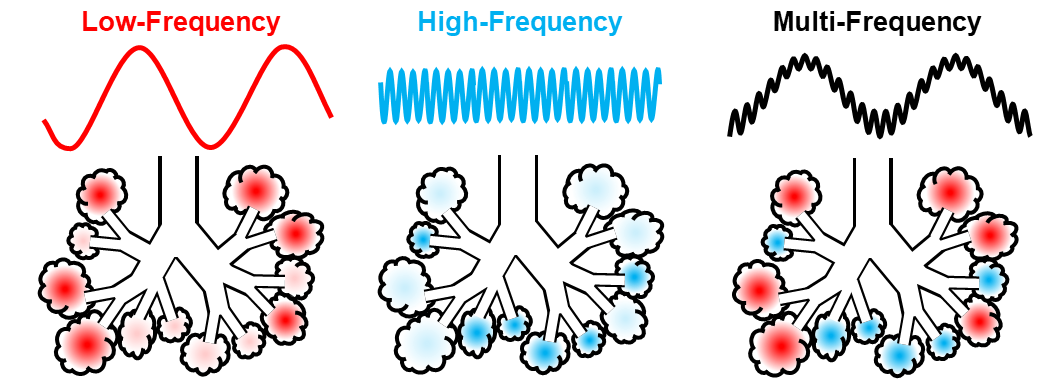
Multi-Frequency Ventilation
Injured lungs are mechanically heterogeneous, such that the distribution of ventilation is also heterogeneous — and highly dependent on the choice of ventilation frequency. Some lung regions may be most ventilated at low frequencies but poorly ventilated at high frequencies, and vice versa. We designed a modality of mechanical ventilation specifically intended to engage different lung regions with different mechanical properties using a combination of multiple oscillation frequencies delivered simultaneously.
This work led to the development of a startup company, OscillaVent, Inc, which is developing and commercializing a prototype ventilator device.
Learn more: Go to PubMed